The equipotential titration curve of virus particles is used to guide the development of ion exchange chromatography
S. Herzer 1 , P. Beckett 1 , T. Wegman 2 , and P. Moore 1
1 Amersham Biosciences Corp, Piscataway, NJ, USA; 2 Mayo Clinic, Rochester, MN, USA
The use of electrophoretic titration curves (ETC) to determine the charge properties of viral particles is part of the chromatographic purification process. The CyDyeTM labeling of viral particles and their detection in gels using the TyphoonTM scanner is a fast and sensitive tool for predicting the chromatographic behavior of intact viruses on ion exchange columns. The ETC results contribute to the choice of ion exchange medium and separation conditions. This article reports the results of adenovirus and measles virus. The method is also applicable to adeno-associated virus (AAV), murine leukemia virus, and bacteriophage such as lambda and M13.
Foreword
Electrophoretic titration curves (ETC) are powerful tools for determining the charge properties of biomolecules in a preset pH range. ETCs for protein mixtures have a large number of references [1-10] and they are commonly used for the evaluation and prediction of ion exchange chromatography [1, 7] .
The requirement of gene therapy and vaccine for a large number of high-purity virus particles has made chromatography separation and purification more and more attention [11-14] . The complexity and friability of viral particles affect the estimation of their charge properties in experiments. Agarose gel electrophoresis in a pH gradient has the advantage of a relatively mild environment. We describe here a fast, reproducible, and sensitive method for determining a useful working pH for chromatographic separation of intact viral particles.
material
All materials, including bacteriophage, were obtained from Amersham Biosciences, unless otherwise stated. The general chemicals used to prepare buffers were from Sigma, Aldrich. SYPROTM Ruby is from Molecular Probes. All other viruses were from the ATCC, except for the measles virus (a friendly donation by Professor M. Federspiel of the Mayo Clinic in Rochester, MN).
method
CyDyeTM fluorescent labeling is done in a weakly alkaline pH environment that maintains virus stability. To avoid over-labeling and cross-linking, only single reactive dyes are used. The marking environment is maintained at a low temperature (on ice) or in a short period of time to ensure that the surface charge properties of the viral particles are not affected by over-labeling.
All other steps are performed in accordance with the manufacturer's instructions, unless otherwise stated. 5% glycerol was added to the agarose ETC gel to prevent agglutination, which showed a large dispersion on the scanned gel.
Run the agarose ETC gel on the PhastSystemTM Rapid Gel Electrophoresis System as follows: First, at 110V, 20 mA/gel, 15 , 7 W °C, 110Vh to form a pH gradient; stop electrophoresis at 110 Vh , rotate the gel 90°. Carefully mark the direction of the cathode during the first and second steps. Samples containing only intact salt-removed viral particles or cell lysates were applied using a titration curve applicator or small incision across the width and pH gradient of the gel. The virus samples were separated by charge/pH at 40-60 Vh at 1000 V 20 mA/gel, 15 , 7 W °C. The separation process is scanned at intervals with an appropriately set Typhoon scanner.
The agarose ETC gel was run on a fast electrophoresis apparatus according to the manufacturer's instructions and observed on a Typhoon scanner with SYPRO Ruby.
Chromatography uses the HiTrapTM IEX Selection Kit, RESOURCETM S and RESOURCE Q columns to separate virus particles. The pH determined according to ETC was chosen for separation and the desalted or diluted virus (appropriate pH at > 5 mS/cm) was separated using a well-balanced column. In general, the column is equilibrated in a low intensity buffer (buffer A, such as 25-50 mM Tris at the appropriate pH) and loaded with 1-3 column volumes (CV) of virus.
Wash the column with at least 1-2 times CV and elute the virus with 10 times CV Buffer A and a gradient to 100% Buffer B, where Buffer B contains the appropriate salt to ensure virus stability, such as 1 M NaCl (consideration compatible) Sex). The components were collected at 0.25-0.5 CV throughout.
The collected fractions were analyzed according to the infectivity of the virus or the Triccorn 5/10 column or MicroSpinTM S-400 HR Column (27-5140-01) using SepharoseTM 6 Fast Flow (17-0159-01). The collected external water volume was analyzed by gel electrophoresis to determine the virus binding characteristics.
Results and discussion
To ensure that the CyDye label does not have a large effect on viral charge behavior, the two tested viral vectors were also isolated on agarose ETC before being labeled. The gel was stained [15] and only mild changes were observed in the migration mode (data not shown).
Agarose gel electrophoresis of CyTM5-labeled adenovirus (AV) showed that AV has a negative surface on the whole pH range of 5 to 10 and a positive charge at pH below 5. 1). This is consistent with the isoelectric point of the quaternary, penta, and fibrin that make up the adenovirus [16] . To determine the pH range that would aid purification, unlabeled starting materials were also separated by electrophoresis and stained with SYPRO Ruby (data not shown).
The virus was isolated from the crude culture on Q Sepharose XL with a pH of 8 (Figure 2). Lower pH values ​​can be used; however, binding capacity will be affected.
At a pH of 8, up to 300 mM NaCl can be used in Buffer A, but this will reduce the binding capacity of the column by half compared to the separation in the absence of NaCl in Buffer A (data not shown).
Figure 1. Adenovirus-tagged Cy5 at 2% agarose
IEF gel, agarose with a pH gradient of 3-10
Gel electropherogram. Anion and cation exchange
Don't be the equipotential point of AIX, CIX) and possible
The pH range is shown in the figure.
Column: Q Sepharose XL, placed on an XK 16/10 column
Sample: Recombinant adenovirus Ad5 CMV-GFP
Sample volume: 5 ml, placed in buffer A
Buffer A: 50 mM TrisCl (pH 8.0), 5% glycerol
Buffer B: Buffer A+1M NaCl
Gradient: 0-100% buffer B, 20 CV
Flow rate: 150 cm/h (5 ml/min) injection 30 cm/h (1 ml/min)
System: ÄKTAexplorer 10
Detection wavelength: 260 and 280 nm
Figure 2. Separation of adenoviruses in cell lysates using anion exchange. The lysate was treated with BenzonaseTM endonuclease prior to loading. The arrow is the adenovirus peak. Its purity is comparable to the two purifications using CsCl centrifugation.

Figure 3. UV inactivation of Cy3 marker measles virus
On a 2% agarose gel with a pH gradient of 3-10
Separation, pI, pH and loading tank
Shown.
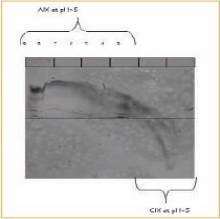
Figure 4. ETC in the pH range of 3-9
Analysis of virus samples in IEF gels.
The gel is stained with SYPRO Ruby
Electrophoresis belts were taken with a Typhoon scanner
Detection.
The stability of type 5 AV using capillary isoelectric focusing has been described [17] . However, this is the first time described to determine the charge behavior of intact viral particles using an electrophoretic titration curve.
The measles virus inactivated by 320 nm UV irradiation for 15 minutes was also analyzed by ETC. The agarose gel electrophoresis curve confirmed that the virus was positively charged at pH below 7, and negatively charged at pH above 7 (Figure 3). However, at pH values ​​above 7, there is a continuing problem of aggregation. Based on experiments with uninactivated measles virus at pH values ​​above 7, aggregation problems appear to be common and are not due to UV irradiation treatment. However, UV crosslinking may increase aggregation.
According to the results of ETC, the measles virus was isolated by cation exchange method. This method selection was also confirmed by electrophoretic analysis of contaminants, and electrophoretic analysis confirmed that a large number of contaminants were negatively charged at pH > 5.5 (Fig. 4). This is somewhat surprising because the isoelectric point of the two envelope proteins of the measles virus indicates that the virus should be negatively charged at pH values ​​above 5.5 [18-20] . These observations indicate the usefulness of determining charge properties based on experience rather than on protein sequences or individual protein isoelectric points. On this basis, SP Sepharose XL successfully purified the measles virus using a pH of 6.5 (Fig. 5).
Ion exchange chromatography can also be applied to the purification of adeno-associated virus (AAV), murine leukemia virus, and bacteriophage such as lambda and M13 (data not shown). Analysis of AAV showed that the overall net charge was negative at pH above 7, and the overall net charge was positive at below neutral pH. Contaminants appear to be negatively charged at pH values ​​above 5 and 5.5. AAV was purified from cell lysates using SP Sepharose High Performance, and purification with SOURCETM 30 Q was better [21].
Moloney mouse leukemia virus exhibits an overall net negative charge at pH above 6, and a net positive charge at pH below 6. This is consistent with the isoelectric point of the surface proteins that have been described (22–25). At above and below that pH, the charge is rapidly converted at a rapidly increasing offset speed. Contaminants appear to be predominantly negatively charged at pH values ​​above 5–5.5, however, the charge is slightly increased over a pH range of 5.5–8. Anion exchange chromatography on RESOURCE Q was used to separate MLV from its major contaminants (data not shown).
Column: Q Sepharose XL HR 5/5 column
Sample: Inactivated measles virus
Injection volume: 2.4 ml, placed in buffer A
Buffer A: 25 mM sodium phosphate (pH 6.5)
Buffer B: Buffer A+1M NaCl
Gradient: 0-100% Buffer B in Step 1
Flow rate: 75 cm/h (0.25 ml/min)
System: ÄKTAexplorer 10
Detection wavelength: 260 and 280 nm
Figure 5. Isolation of UV-inactivated measles virus in SP Sepharose XL at pH 6.5. The black line shows the virus-containing fraction (based on gel filtration analysis data).
in conclusion
Electrophoretic titration curves are a simple, rapid method for the preliminary screening of ion exchange chromatography conditions for viral particles. The method described herein relies on CyDye labeling and observation using a Typhoon scanner for rapid and sensitive detection of viral particles. As noted above, ETC is used to determine an appropriate pH range for ion exchange chromatography of intact viral particles. In some cases, only empirical data generated by ETC electrophoresis titration can be relied upon to determine the appropriate pH range, and the isoelectric point of published capsid proteins is not suitable for determining viral purification conditions.
references
1. Lindblom, H. et al. Separation of urine proteins on the anion-exchange resin mono QJ Chromatogr. 273, 107–116 (1983).
2. Nath, S. et al. Correlation of migration behavior in free-flow zone electrophoresis and electrophoretic titration curve. Electrophoresis, 11, 612–616 (1990).
3. Avellana-Adalid, V. et al. Electrophoretic study of conformational changes of a human soluble beta-D-galactoside-binding lectin upon storage. Electrophoresis, 13, 416–421 (1992).
4. Hull, HH and Wharton, DC Isoelectrophoretic characterization of Pseudomonas cytochrome oxidase/nitrite reductase and its heme d1-containing domain. Arch. Biochem. Biophys. 301, 85–93 (1993).
5. Brisabois, A. and Gullet, P. Isolation and characterization of carboxylesterase E3 from Salmonella enterica. J. Appl. Bacteriol. 75, 176-183 (1993).
6. Picard, B. et al. Genetic heterogeneity of Pseudomonas aeruginosa clinical isolates revealed by esterase electrophoretic polymorphism and restriction fragment length polymorphism of the ribosomal RNA gene region. J.Med. Microbiol. 40, 313–322 (1994).
7. Watanabe, E. et al. Selection of chromatographic protein purification operations based on physicochemical properties. Ann. NY Acad. Sci., 721, 348-364 (1994).
8. Attanasio, F. et al. Analytical titration curves of glycosyl hydrolase Cel45 by combined isoelectric focusing-electrophoresis. Electrophoresis, 20, 1403-1411 (1999).
9. Ameskamp, ​​N. et al. Pilot scale recovery of monoclonal antibodies by expanded bed ion exchange adsorption. Bioseparation 8, 169-188 (1999).
10. Sanchez, EE et al. Partial characterization of a basic protein from Crotalus molossus molossus (northern blacktail rattlesnake) venom and production of a monoclonal antibody. Toxicon 39, 523-529 (2001).
11. O'Riordan, CA et al. Scaleable chromatographic purification process for recombinant adeno-associated virus (rAAV). J. Gene Med. 2, 444-454 (2000).
12. Gao, G. et al. Purification of recombinant adeno-associated virus vectors by column chromatography and its performance in vivo. Hum. Gene Ther. 11, 2079-2091 (2000).
13. Tamayose, K., et al. A new strategy for large-scale preparation of high-titer recombinant adeno-associated virus vectors by using packaging cell lines and sulfonated cellulose column chromatography. Hum. Gene Ther. 7, 507-513 ( 1996).
14. Huyghe, BG et al. Purification of a type 5 recombinant adenovirus encoding human p53 by column chromatography. Hum. Gene Ther. 11, 1403-1416 (1995).
15. Westermeier, R. Electrophoresis in Practice, 3rd ed., WILEY-VCH Verlag GmbH, Weinheim, (2001).
16. Adam, E. et al. comparative studies on the soluble proteins of adenovirus type 1. Acta Microbiol Acad Sci Hung. 24, 181-187 (1977).
17. Mann, B. et al. Capillary zone electrophoresis of a recombinant adenovirus. J. Chromatogr. A 895, 329-337 (2000).
18. Kohama, T. et al. Maturation of measles virus hemagglutinin glycoprotein. Arch. Virol. 85, 257-268 (1985).
19. Boriskin, YuS. et al. Measles virus persistent infection: modification of the virus nucleocapsid protein. J. Gen. Virol. 67, 1979-1985 (1986).
20. Andzhaparidze, OG et al. Analysis of the possible mechanisms for the persistence of the vaccinal strain of the measles virus in a human cell culture. Vopr Virosol. 33, 338-342 (1988).
21. Brument, N. et al. A versatile and scalable two-step ion-exchange chromatography process for the purification of recombinant adeno-associated virus serotypes-2 and -5. Mol. Ther. 6, 678-686 (2002).
22. Strand, M. and August, JT Polypeptide maps of cells infected with murine type C leukemia or sarcoma oncovirus. Cell 13, 399-408 (1978).
23. Forchhammer, J. and Turnock, G. Glycoproteins from murine C-type virus are more acidic in virus derived from transformed cells than from nontransformed cells. Virology 88, 177-182 (1978).
24. Katoh, I. et al. Murine leukaemia virus p30 heterogeneity as revealed by two-dimensional gel electrophoresis is not an artefact of the technique. J. Gen. Virol. 65, 733-741 (1984).
25. Ikuta, K. and Luftig, RB Differences in the pI heterogeneity of virion and intracellular Moloney murine leukaemia virus p30s. J. Gen. Virol. 68, 487-498 (1987).
Hyaluronic Acid Meso Serum is the use of hyaluronic acid injections directly on the skin, allowing the facial skin moist and soft, shiny and translucent, so you have artists like smooth and translucent skin, the dermis can solve the problem of lack of water, improvement of small wrinkles, bring significant results.
It is sterile, biodegradable, nonpyrogenic, viscoelastic, clear, colorless, homogenized serum consists of hyaluronic acid(HA), formulated to a concentration of 10mg/ml-30mg/ml according to different use.
Following treatment is recommended after 3-4 weeks. It is recommended to repeat the treatment after 6-9 months.
Our Meso Serum can use by Hyaluron Pen, meso gun, derma pen ,etc
For details,welcome to inquiry us,we will provide highly quality,competitive price for you !
Mesotherapy Serum
Mesotherapy Hyaluronic Acid,Hyaluronic Acid Meso Serum,Meso Serum,Mesotherapy Serum
Qufu Hi-Tech Trading Co., Ltd. , https://www.chinahyaluronic-acid.com